Fermi LAT masterclass
Introduction
Gamma-ray astrophysics is one of the youngest branches of astronomy. It enhances our understanding of some of the most violent explosions and extreme objects in space: supermassive black holes, supernova explosions, pulsars, gamma-ray bursts.
In the first part of the masterclass we will give a short introduction into the gamma-ray astrophysics, we will talk about sources of gamma-rays in space and how the gamma-ray emission from these objects is detected.
In the second part of the masterclass we will have hands on exercises aimed to explore the real gamma-ray data collected by the Fermi Large Area Telescope (a detector of gamma-rays on a satellite orbiting around the Earth). We will work in groups. Each group will analyze its own source of gamma rays and make a short report about the source and their work at the end.
Gamma rays
Electro-magnetic radiation propagates in space as waves, such as radio waves, microwaves, or visible light. The waves are described by a frequency 𝛎: this is what one tunes in radio in order to select a radio station, for example, 100 MHz (mega Herz) corresponds to 100 Million oscillations per second. The frequency is inversely proportional to the wavelength 𝛌 = c / 𝛎 – for smaller frequencies the wavelength is larger and vice versa, here c ≈ 300 000 km/s is the speed of light.

One of the biggest wonders of the world is that the waves are emitted and absorbed by discrete quanta with energies proportional to the frequencies: E = hν, where h is Planck’s constant. These discrete quanta of electromagnetic radiation are called photons. Depending on the frequency (or equivalently the energy of the photon) one distinguishes different types of radiation, such as microwaves, visible light, or X-rays. Photons of highest energies are called gamma rays.
Such photons are created for example in radioactive decays of nuclei. In space gamma rays can be produced as a result of collisions of high energy cosmic rays (such as protons or electrons traveling almost at the speed of light) with interstellar gas. Since cosmic rays of highest energies are accelerated in some of the most extreme events in space, such as supernova explosions, collapse of stars in the process of black hole formation, or gas clouds falling onto supermassive black holes, gamma rays produced by these cosmic rays gives us one of the most important windows into the physics of the most extreme events in space.
Gamma rays do not reach the ground due to interactions in the atmosphere. Consequently, one detects gamma rays either by observing the showers of particles created by the gamma ray in the atmosphere (this is possible for gamma rays of very high energy) or by placing the detector above the atmosphere on a satellite. Fermi Large Area Telescope is a detector of gamma rays on a satellite.
The Fermi Large Area Telescope (LAT)

Fermi LAT gamma-ray space telescope is a detector of gamma rays on a satellite 530 km above the ground.
Launched in 2008.
Makes one rotation around the Earth in about 45 mins.
Consumes only about 650 W of power (similar to a toaster).
International Collaboration: 400 scientists and students at more than 90 universities and laboratories in 12 countries.

The Fermi LAT detector consists of three parts:
Tracker
An incoming gamma ray produces an electron-positron pair (positron is the antiparticle of the electron) – this process is called ‘conversion’. The trajectories of the electron and positron are recorded by the “tracker” part of the detector. These trajectories are used to determine the direction of the incoming gamma ray.
Calorimeter
The energy of the electron-positron pair is deposited here. This energy is used to reconstruct the energy of the incoming gamma ray.
Anti-Coincidence Detector
This part of the instrument is used to separate the gamma rays from charged cosmic rays, such as electrons and protons. It consists of scintillating plates which make a flash of light when a charged particle goes through, but remain dark for gamma rays.
Sources of gamma rays

http://cds.cern.ch/journal/CERNBulletin/2009/28/News%20Articles/1187591?ln=en
This map shows about three months of all-sky data collected by the Fermi LAT. The bright yellow-red band in the middle is the plane of our Galaxy (the Milky Way). Most of Galactic sources (such as pulsars and supernova remnants) are found in the plane. Most of sources outside of the plane are extragalactic, such as active galactic nuclei and gamma-ray emission from other galaxies. We will give a short description of various sources of gamma rays below.
Sources of gamma rays in our Galaxy
Our Solar system is located inside the Milky Way galaxy. In our Galaxy, which is of the “spiral” type, there are hundreds of billions of stars. Some of these emit gamma radiation and are therefore detectable by Fermi. The two most numerous sources of gamma rays in our Galaxy are pulsars and supernova remnants.
Supernova Remnants
Supernova Remnants (SNRs) are galactic sources created by supernova explosions. Massive stars at the end of their life do not produce enough energy to counteract the gravitational attraction of the gas towards the center of the star. As a result, the star collapses, which results in the release of the gravitational energy as an explosion (these are called core collapse supernovae). As a result of the explosion there is an expanding shell and a very dense remnant in the center, e.g., a neutron star (discussed below). SNRs are extremely important for understanding our galaxy. They heat up the interstellar medium, produce heavy elements , and accelerate cosmic rays.

The Crab Nebula is a supernova remnant and pulsar wind nebula in the constellation of Taurus. The common name comes from William Parsons, 3rd Earl of Rosse, who observed the object in 1840 using a 36-inch telescope and produced a drawing that looked somewhat like a crab. The nebula was discovered by English astronomer John Bevis in 1731, and it corresponds with a bright supernova recorded by Chinese astronomers in 1054. The nebula was the first astronomical object identified that corresponds with a historical supernova explosion.
The filaments on the outside of the Crab nebula are the remains of the expanding shell produced by the supernova explosion in 1054. At the center there is also a pulsar detected, the Crab pulsar. The bluish haze in the middle is believed to be the pulsar wind nebula, inflated by the high energy electrons and positrons created and accelerated by the pulsar. The gamma-ray emission from the Crab pulsar and the corresponding nebula can be located on the gamma-ray map above in the Galactic plane on the far right.
Pulsars
As a result of the core collapse supernova explosion, there is a very dense remnant in the center with a mass between 1.5 and 2 masses of the Sun and a radius of about 10 km.This very dense object consists of primarily neutrons and for that reason called a neutron star. They are the densest known objects consisting of normal matter (thus excluding black holes). A few characteristic of neutron stars:
- Density comparable to the density in atomic nucleus: a tea-spoon filled with a matter of such density would weigh about 1 billion tons;
- very strong magnetic field on the surface: it is 10 million times larger than the magnetic field used in the Large Hadron Collider in CERN or trillion times larger than the magnetic field of the Earth;
- short period of rotation: right after the formation neutron stars rotate a few hundred times per second.
Due to fast rotation and large magnetic fields, pulsars electron-positron pairs are created and accelerated to very high energies. These electron-positron pairs together with electrons ripped of from the surface of the neutron stars emit radiation at different frequencies from radio to high energy gamma rays. This radiation is beamed and we observe the rotating neutron stars as pulsating objects, e.g, pulsars, due to the lighthouse effect. With time pulsars loose rotational energy due to emission of radio waves and creation and acceleration of electron-positron pairs. As a result, depending on the age of the pulsar, it can have the frequency of rotation from a few hundred rotations per second up to one rotation in several seconds.
Pulsars were discovered when a graduate student at the University of Cambridge, England, named Jocelyn Bell was poring over the data from her radio telescope – 120 meters of paper recordings. She found something peculiar about these observations: They seemed to be periodic with a period of 1.33730 seconds. This was the very first pulsar discovered, known today as PSR B1919+21, with the markings being called ‘Little Green men’, since they were thought to be signals from extraterrestrial life at first. This pulsar is simulated below (taken from https://nasa.tumblr.com/post/163637443034/five-famous-pulsars-from-the-past-50-years ).
Cool Fact: This is the pulsar on the cover of Joy Divisions Album “Unknown Pleasures”!
Millisecond Pulsars
There is a subtype of pulsars with very small periods of rotation – a few milliseconds – called millisecond pulsars (MSPs).
An MSP is a pulsar with a rotational period smaller than about 10 milliseconds. Millisecond pulsars have been detected in radio, X-ray, and gamma ray parts of the electromagnetic spectrum. The leading theory for the origin of millisecond pulsars is that they are old, rapidly rotating neutron stars that have been spun up or “recycled” through accretion of matter from a companion star in a close binary system. For this reason, millisecond pulsars are sometimes called recycled pulsars (see video at: https://sci.esa.int/web/integral/-/52867-an-ordinary-pulsar-evolving-into-a-millisecond-pulsar). The difference with young pulsars, which also have periods of rotation of a few millisecond is that the magnetic field of MSPs is about 10 thousand time smaller, as a result, they loose their energy much slower than the young pulsars and can continue rotating with the very small periods for billions of years.
Extragalactic sources of gamma rays
Apart from sources inside of the Galaxy, gamma ray emission is observed from extragalactic sources, i.e., sources outside of our Galaxy. Two main types of such sources are Active Galactic Nuclei (AGNs) and Gamma-ray Bursts. AGNs in particular outnumber the known pulsars and other sources in the Fermi LAT catalog.
Active galactic nuclei
The cores of many galaxies produce enormous amounts of radiation at all wavelengths. These Active Galactic Nuclei or AGN are assumed to be fueled by accretion of matter onto supermassive black holes with masses ranging from millions to billions times the mass of the Sun (solar mass). The high energy emission is thought to be released in a corona of hot material in the accretion disk and/or in powerful jets. This is shown in the schematic below:
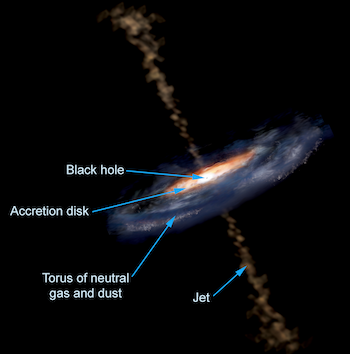
Gamma-ray bursts
Gamma-ray bursts (GRBs) are short-lived bursts of gamma-rays. They last between tens of milliseconds to several minutes. GRBs shine hundreds of times brighter than a typical supernova and about a million trillion times brighter than the Sun. When a GRB erupts, it is briefly the brightest source of cosmic gamma rays in the observable Universe.

There are two types of GRBs: long and short. The separation is approximately at the duration of 2 sec (see the plot below). Short duration bursts are believed to be produced by a merger of two neutron start, which results in a creation of a black hole. Long duration bursts are produced when the core of an old massive star collapses (similar to the core collapse supernovae), but the core is so heavy that instead of creation of a neutron star it further collapses to form a black hole.

Further information about various gamma-ray sources and all-sky maps of their distributions derived from Fermi-LAT data can be found on this page.